Pharmacogenomics and Genomics
Examples of department research and methods in this area include
Determining genomic differences underlying asthma prevalence, severity, and treatment response in minority children
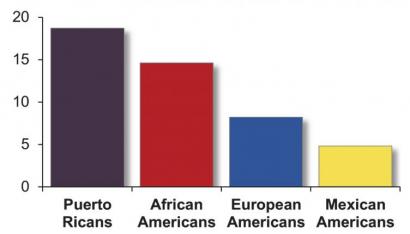
Prevalence of asthma in U.S. children
Asthma, a recurrent inflammation that narrows the airways, is the most common chronic disease in American children. It is roughly twice as prevalent and causes more deaths among Puerto Rican and African American youth than in those of European ancestry, while Mexican American children exhibit a relatively low prevalence. In addition, the most commonly prescribed asthma medication (the inhaled bronchodilator albuterol) is significantly less effective in Puerto Ricans and African Americans.
Discovering why this is the case has been stymied by the lack of representation of these minorities in genome-wide association study (GWAS) populations—fewer than 4 percent of such studies have been conducted in non-Europeans.
Department scientists lead the largest study of the interactive effects of genetics, environmental exposures, and socioeconomic factors on asthma in minority children (Latino- and African-Americans) in the United States, a multi-center effort with a total of more than 9,000 participants. Such research has found that 82 percent of genes associated with asthma in other racial/ethnic populations were not associated in Latinos (Puerto Ricans, Mexicans).
In addition to GWAS to identify genomic variants associated with disease prevalence, severity, and drug response, researchers here apply complementary methods that include:
-
Ancestry admixture mapping—which uses genetic markers and statistical software to infer whether a genome region was passed from African, European, or Native American ancestors. The ancestral diversity among admixed study populations such as Latinos (modern descendants of all three groups) can thus be leveraged to detect regions associated with disease risk and drug response. For example, department research has found Native American ancestry in one region (HLA on chromosome six) protects against asthma in Latinos, while at another genetic locus (SMAD2) it is associated with increased risk of the disease.
- Candidate genome loci sequencing—which more closely examines variants implicated through GWAS and admixture mapping for asthma susceptibility, as well as for bronchodilator drug response and related traits such as blood serum levels of immunoglobulin E (IgE), a class of antibodies elevated in allergic disorders. For example, research here deep-sequenced seven such genes in 2,000 Latinos, both their protein-encoding exons as well as untranslated regions (UTRs), which regulate messenger RNA (mRNA) and thus functional protein expression, to discover new variants within three of the genes significantly associated with drug response and total IgE.
Department researchers also study how such genetic variation significantly interacts with environmental and socio-economic conditions, ranging from childhood exposure to specific components of outdoor air pollution, and from secondhand to in utero tobacco smoke exposure (including, in the latter, potential DNA modification via methylation), and socioeconomic status.
Characterizing mutations in gene regulatory elements as causes for disease and differences in drug response
Genes actually make up only 2 percent of our DNA. Within the remaining 98 percent there are extremely important nucleotide sequences that function as gene regulatory elements. These are segments of DNA that instruct genes when, where, and to what extent to turn on or off. Department scientists are investigating whether mutations in these regulatory elements are a significant cause of human diseases such as epilepsy, limb malformations, obesity, autism, scoliosis, and hernia, and if they also lead to differences in how we respond to drugs.

Gene regulatory elements are activated when proteins called transcription factors bind to them. Categorized by their effect on genes, they include:
- Promoters—serve as the site where enzymes and other proteins bind and assemble to start making RNA from DNA, the first step in gene expression (i.e., the creation of protein molecules).
- Enhancers—activate promoters at specific locations, times, and levels.
- Silencers—turn off gene expression at specific locations, and times.
- Insulators— prevent one gene’s regulatory elements from affecting its neighbors.
One such research area is epilepsy, which is a group of disorders characterized by recurrent unprovoked seizures. While it is one of the most common neurological disorders, affecting more than 60 million worldwide, in the majority of cases its cause is unknown. Indeed, the disease’s complexity and variation can make it difficult to precisely diagnose and effectively treat.
Researchers here, along with international collaborators, are examining whether mutations in those elements that regulate genes involved in brain development lead to epilepsy sub-types. (A prime example is infantile spasms, a seizure disorder that typically starts in the first year of life and can interfere with normal development.)
To identify those mutations, researchers here are first determining which gene regulatory elements affect developmental expression of about 100 different genes that have been linked to epilepsy. They are analyzing DNA from the forebrain of a mouse embryo, which is genetically equivalent to the human brain region that is the source of seizures in infantile spasms. This is done using antibodies and deep sequencing techniques to detect active enhancers—regulatory elements that kick-start gene activation, by activating other elements called promoters—of the epilepsy-related genes. Those findings are confirmed by injecting the enhancer-promoter pairings into animal models linked with reporter genes that express glowing proteins if an epilepsy-related gene is activated during brain development. Genomes from patients with infantile spasms are analyzed for mutations in those enhancers, which are then assessed for changes in function in animal models.
The goal is to provide a rapid, genome-based diagnosis of epilepsy sub-types such as infantile spasms to better guide therapies and to develop a model for determining the roles of gene regulatory elements in other complex diseases.
Nucleotide variation in regulatory elements can also have a significant effect on drug response; potentially, for example, altering genetic expression of drug metabolizing enzymes and/or transporter proteins, which regulate drug passage into target tissues, organs, and cells. But these elements have been understudied because it can be difficult to identify them. For example, enhancer sequences are not necessarily located near the promoters and genes they activate, but can be hundreds of thousands of base pairs away. (Activated enhancers may interact with promoters via changes in DNA shape.)
Department scientists are using various genomic assays (RNA-seq, ChIP-seq, ChIA-PET) to analyze human liver cells treated with drugs compared to controls to identify drug-activated regulatory elements genome-wide. They then test these elements for the activity in human liver cells with and without drug and analyze drug-activated sequences to see whether variations in them alter their activity.
Combined, such work identifies gene regulatory elements that can be activated due to drug response and provides candidate sequences where nucleotide variation can lead to differences in drug response.
Determining genetic differences in response to the most widely used diabetes drug in ethnically diverse populations
Type 2 diabetes, in which the body is less able to use the hormone insulin to regulate blood sugar (glucose), affects 350 million patients globally. In the United States, it is the leading cause of blindness, kidney failure, and non-accident related amputations. Since 1980, the number of U.S. adults with diabetes has risen five-fold to 29 million, or 9.3 percent of the population.
Metformin is the recommended, most widely used initial medication for type 2 diabetes and one of the most prescribed drugs worldwide. But there is substantial variation in response among individuals. More than one-third of patients do not achieve blood sugar control from the drug alone or at all.
Department scientists seek to discover the genetic differences underlying those major differences in metformin response. Their approaches include:
Using pharmacokinetics as a guide
Put simply, pharmacokinetics is what the body does to a drug (absorption, distribution, metabolism, excretion) and pharmacodynamics is what a drug does to the body (therapeutically altering biological activities within or between cells).
Metformin appears to work mainly by altering the insulin sensitivity of liver cells, reducing their glucose production. The drug is not metabolized; rather it is eventually excreted intact. Thus research here has focused on genetic differences in how the body handles metformin, regulating its rate of absorption into the liver cells where it acts and its rate of excretion via the kidneys into urine.
Discovering key transporters
Department scientists have focused on transporters—proteins in cell membranes that regulate the passage of molecules, including drugs, into and out of cells. Researchers here were among those who found, initially in cell cultures and animal models, that a transporter called organic cation transporter 1 (OCT1) was crucial to metformin’s absorption into liver cells (hepatocytes) and that variations in that protein altered the drug’s effects in studies of both healthy human volunteers and diabetic patients. Several other transporters were also found to play major roles in clearance of metformin from the body via absorption into the kidney (OCT2) and excretion in urine (multidrug and toxin extrusion proteins1 and 2, or MATE1 and MATE2).
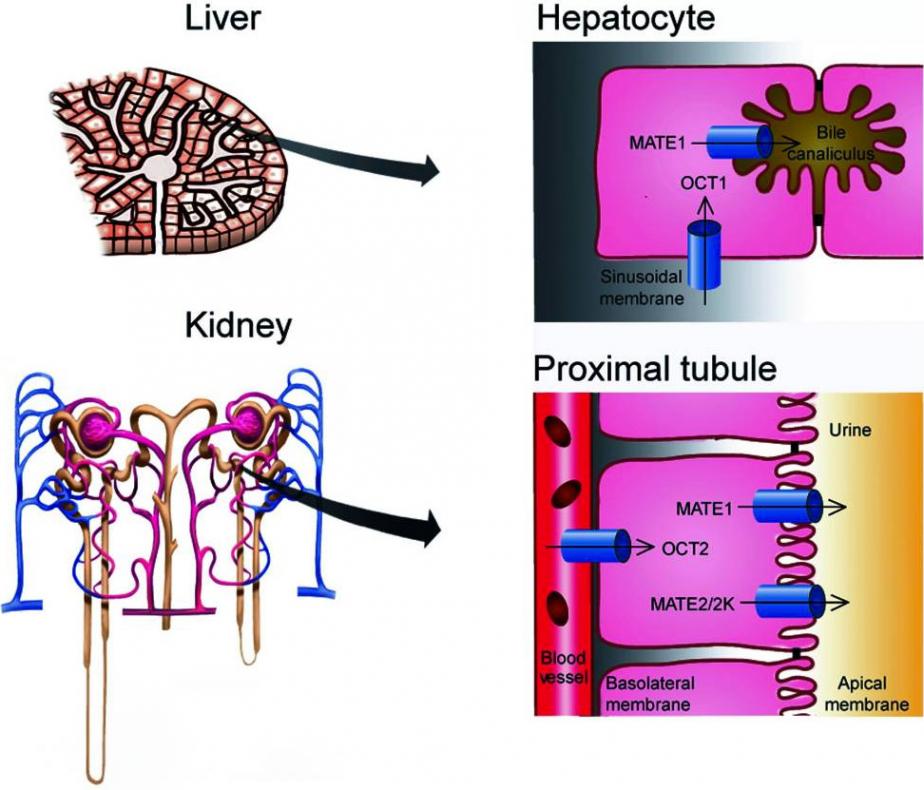
Schematic of transporter proteins in liver hepatocyte cells and kidney proximal tubule cells that have been identified as important determinants of metformin pharmacokinetics and response.
Analyzing diverse populations
To determine the genetic variations giving rise to those differences in transporter uptake and efflux of metformin, department scientists have conducted genome-wide association studies of ethnically diverse groups of healthy volunteers and diabetic patients, associating SNP variants with drug response and clearance rates.
Study population diversity is vital because there are major differences in the type and frequency of transporter genetic variations among Caucasians, Asians, Latinos, and African-Americans. The department is home to the on-going Study of Pharmacogenetics in Ethnically Diverse Populations (SOPHIE) study, which provides healthy controls, and researchers here tap genomic data from the department-based National Institutes of Health-funded Pharmacogenetics of Membrane Transporters Database and the international 1000 Genomes Project.
Focusing on gene promoters
In addition to variations in the genes that generate (express) the key transporters, research here has discovered variations in promoters, the non-coding regulatory elements adjacent to those genes that control their levels of expression (See illustration in section above.). These variants help determine metformin clearance and anti-diabetic response in both patients and healthy volunteers.
Looking upstream to transcription factors
Given that overall absorption, distribution, and excretion of metformin is controlled by several transporters in the liver and kidney, each variation in a transporter-encoding gene accounts for only a fraction of the differences in patient drug response. So department scientists led the first study looking upstream at variations in genes expressing transcription factors—proteins that activate or suppress genes by binding to gene regulatory elements—which may, in turn, regulate the expression levels of multiple genes that encode a combination of metformin-relevant transporters to greater effect. Correlating the genomic and clinical data of hundreds of patients and healthy volunteers, the department-led study found genetic variants in specific transcription factors that significantly affected their drug responses and/or clearance rates.
Determining how genetic differences alter response to the primary drug for preventing gout
The prevalence of gout, a severely painful inflammatory arthritis caused by the crystallization of excess uric acid in the joints, has been increasing with so-called metabolic syndrome, which includes obesity and hypertension. Gout now affects more than 8 million U.S. adults. Allopurinol is the major medication used to prevent gout flare-ups, but one-half of patients do not respond to the drug.
Department scientists have conducted the first genome-wide association study of allopurinol response. They noted that variations in a genetic locus that codes for ATP Binding Cassette (ABCG2), a membrane transporter protein that regulates the drug’s access to certain areas of the kidney, plays a major role in allopurinol response.
The hypothesis now being tested here is that in addition to inhibiting the enzyme that synthesizes uric acid, allopurinol also inhibits transporters in the kidney that otherwise reabsorb uric acid, but genetic variants in ABCG2 reduces the drug’s access to those transporters.
Dissecting the molecular genetics of liver cancers
Multiple, highly regulated biological pathways—cascading sequences of interacting protein molecules—control cell replication and tissue growth. Cancers can occur when mutations in key genes, including oncogenes or tumor suppressor genes, alter the type or extent of proteins they express, changing those pathways to enable unchecked malignancies.
Liver cancer is one of the most common malignancies globally, accounting for 745,000 deaths worldwide in 2012, second only to lung cancer. Effective treatments are currently lacking.

Diagrams of part of Hippo tumor suppressor pathway, here showing regulation by integrin-linked kinase (ILK). Turned off (left) by ILK, the pathway allows activation of Yap and TAZ, leading to cell proliferation. Turned on (right), the sequence inactivates the two gene coactivators.
Genetic studies support the importance of the Hippo tumor suppressor pathway, which controls organ size and cell proliferation. (Hippo refers to a key pathway enzyme, Hippo kinase—mutations in the gene that expresses it in drosophila allows tissue overgrowth, akin to a hippopotamus.) Indeed, in a mouse deletion model, it has been shown that inactivation of Hippo kinases led to increased cell proliferation, liver stem cell expansion, and eventually tumor development.
Department scientists have found that two coactivator proteins called Yap and TAZ, which are normally inactivated in the Hippo pathway, are over-expressed and activated in a subset of human liver cancers, and synergize with another signaling pathway to accelerate liver cancer development in mice. (Coactivators increase gene expression by binding to another activator or transcription factor.)
Researchers here are analyzing the roles of Yap and TAZ during liver cancer development to determine the molecular mechanisms underlying the acceleration of tumor development induced by co-expression of these proteins with other cancer-associated signaling pathways (AKT, Ras, and c-Myc). They are using conditional knockout mouse models, in which Yap- and TAZ-expressing genes are deleted specifically in liver cells, to study the requirement of these co-activators in those pathways’ tumor development. The goal is to understand these oncogene activators in order to target them with new drugs.